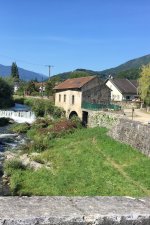
France Collection Site
This photo taken in the mountain regions of France shows one of many Podarcis muralis collection sites we will be using in our Theory-to-Practice grant next summer to continue our research.
Adapting to the environment is the driving force behind life on Earth. Perhaps one of the most difficult challenges all organisms face is that of thermoregulation. Thermoregulation is an organism’s attempt to maintain their preferred body temperature within their environment. Organisms that rely on the surrounding environment for their thermoregulation are deemed as ectothermic, or more commonly known as “cold-blooded”. However, due to their dependencies on the environment to maintain body temperature, ectotherms have little thermal tolerance buffer, and must rely largely on physiological, and behavioral strategies for thermoregulation.
Due to climate change, the environments on Earth are fluctuating to a significant degree, and the organisms living in these changing environments must adapt or risk being wiped out. Due to their dependence on the surrounding environment to maintain appropriate body temperatures, populations of ectothermic organisms are especially at high risk of being impacted from climate change. To study the impact of climate change on ectotherms, we will be conducting research in the coming year, supported by a Theory-to-Practice grant, to travel to France to conduct a variety of experiments on the lizard species Podarcis muralis. This ectotherm is an active thermoregulator found in different elevations all along the Pyrennees mountains of France, and has invasive populations in Ohio in the Cincinnati area. We seek to answer questions about how Podarcis muralis’ thermoregulatory strategies, such as regional heterothermy and other behavioral adaptations, are affected by changes in the environment, like elevation differences and percentage of humidity.
Ectothermic organisms depend entirely on the surrounding environment in order to maintain thermal balance. If the environment has low thermal quality, or if they are not in range of their thermal optimum, they must adjust their behavior to compensate. To uncover the mechanisms and driving forces behind lizard thermoregulation, we must first understand how environmental temperatures affect lizards, and how they accordingly respond. In this project, we address questions about the role of humidity and moisture levels in lizard thermoregulation and the impact of the surrounding environment on lizard activity levels. Further, we investigate how regional heterothermy (maintaining different temperatures in different regions of the body) fluctuates in a changing environment. To answer these questions, we will conduct research on the lizard species Podarcis muralis, the Common Wall Lizard. This species is an active diurnal thermoregulator native to Europe, but found in abundance here in southern Ohio. This makes it an ideal organism to conduct experiments studying how ectotherms compensate for environmental changes that impact thermoregulation. We will conduct field transects of natural populations and use 3D printed model lizards to measure operative temperatures in the field. The environment (temperature, humidity, light levels, etc.) and subject (body and cloacal temperature, activity levels, etc.) will be quantified to test hypotheses that will give us insight on understanding how ectothermic organisms respond and adapt to shifts in the environment. As climate change impacts the Earth, many organisms, especially ectotherms, will need to adjust their thermal interactions with the surrounding environment.
THERMOREGULATION, THERMAL MODELLING, ECTOTHERMS, HUMIDITY, REGIONAL HETEROTHERMY
Data of body, head, and cloaca temperature from both the live lizard (François) and the 3D model (Charizard) over time. The red line bisecting this graph indicates when the heat source was removed, marking the beginning of the cooling period.
To measure the thermoregulatory behavior and effectiveness of an organism, it is necessary to record body temperatures of individual animals at the same time as temperatures in an animal not thermoregulating. The most common way to do this is to deploy model animals that mimic living animals in their thermal qualities, but don’t move. We are utilizing 3D printed model lizards as our operative models in field studies, but first need to compare the thermal qualities of these plastic models against a living animal. We simultaneously tested the thermal properties of our 3D printed model lizard and a living animal. We first slowly heated them both in the same environment to an optimal temperature (36℃) and then allowed them to slowly cool. During this time, we used a thermal imaging camera to record surface temperature and a thermal probe to record internal body temperature of both subjects at set intervals. We predicted that the 3D model and the live lizard would have similar rates of thermoregulation, as the model was built to mimic the thermal properties of the live Podarcis muralis. Our second prediction was that the live lizard would exhibit regional heterothermy (a physiological strategy where the ectotherm maintains different temperatures in different regions of the body) as there have been reports of P. muralis using this strategy.
This experiment has helped us prepare for our Theory to Practice Grant to study P. muralis in France by introducing us to experimental design, initiating us into the research process, teaching us project and time management, and giving us experience on the responsible and appropriate handling and monitoring of live test subjects.
Once our experiment was completed, statistical tests were run on our data using the programming language R. For the first half of the experiment, during the heating phase, the body temperature of the 3D model (designated as Charizard) heated up faster than the body of the live lizard (designated as François). When comparing the data between the two, an adjusted R2 value of 0.219 was reported, indicating that the body temperatures of the two subjects are significantly correlated, but this correlation is not very strong.
This is reflected in the graph, as the body temperature of the 3D printed model clearly jumps to peak temperature within the first 5-10 minutes, while the live lizard gradually increases, reaching its peak at the 25-30 minute mark. The cooling period however, shows a different trend. Once the heat source is shut off and the cooling period begins, the body temperature of the 3D model begins to follow the live lizards cooling patterns. The data for this period reflects this, as the adjusted R2 value changes, now reporting a much stronger correlation of 0.886. One possible explanation for these results could be due to the material, size, or density of the 3D model not being an 100% match for the thermal qualities of the live lizard. Our next step is to build a model slightly smaller in size with a more dense plastic to better match the thermal qualities of a live animal.
As for the second hypothesis, regarding the presence of regional heterothermy within P. muralis , the statistical data and analysis confirmed our suspicions. In the heating phase of this experiment, the adjusted R2 value was reported as 0.988. This value, being very, very close to 1.00, tells us that the temperature in the body and in the head had a very strong correlation with one another. However, as seen on the graph, at the 20 minute mark, the body and head temperatures begin to diverge, and this divergence continues until the end of the cooling stage. The statistics support this, with a R2 value at 0.834, a respectable difference from the previous 0.988 value seen during the heating phase. Taken together, this indicates that the patterns did diverge from each other to a significant degree, confirming our second hypothesis.
In conclusion, the 3D model and the living lizard were not a perfect match, but our data will help inform our next steps in model construction. Furthermore, regional heterothermy was evident within the P. muralis individual suggesting a potentially interesting avenue for research, such as studying how different types of environmental manipulation, such as terrain differences, seasonal timing, or nutrition could further impact regional heterothermy within an individual.
We are grateful for assistance in creating the 3D printed model lizard from Dr. Chris Wolverton and Joseph Gangloff. This project has received funding support from TPG and TEW grants from Ohio Wesleyan University.
This photo taken in the mountain regions of France shows one of many Podarcis muralis collection sites we will be using in our Theory-to-Practice grant next summer to continue our research.